Trace amines (TAs) are endogenous biogenic amines and closely related to biogenic amine neurotransmitters. They are formed by decarboxylation of aromatic amino acids and are only present in small amounts in vertebrates (order of magnitude 1/100 of the corresponding neurotransmitters). In invertebrates, however, they are common and are involved in an equivalent of the mammalian adrenergic system and the fight-and-flight response. Invertebrates do not possess TAAR.
Trace amines are involved in the regulation of dopamine, glutamate and serotonin neurotransmitter metabolism in the brain.
In high concentrations, they have a similar effect to amphetamines at the presynapse on the release, reuptake and biosynthesis of catecholamines and indolamines. In low concentrations, they show modulating effects at the postsynapse, which increase the activity of other neurotransmitters, especially dopamine and serotonin.
-
1. Traceamine
-
2. Trace amine receptors
-
2.1. HTAAR1 (TA-1, TAR-1, TRAR-1)
-
2.2. HTAAR2 (GPR58)
-
2.3. HTAAR3 (GPR57P)
-
2.4. HTAAR4 (TA2, TA2P, 5-HT4P)
-
2.5. HTAAR5 (PNR)
-
2.6. HTAAR6 (TA4, TRAR4)
-
2.7. HTAAR7 (TA12, TAAR7a and others)
-
2.8. HTAAR8 (TA5, TRAR5, TAR5, GPR102)
-
2.9. HTAAR9 (TA3, TRAR3, TAR3)
-
3. Degradation of trace amines
-
4. Interaction dopamine system / trace amine system
-
5. Trace amines and ADHD
1. Traceamine¶
1.1. Dopamine-related trace amines¶
- Β-Phenylethylamine
- N-methylphenylethylamine
- Secondary trace amine
- N-methylphenylethylamine derivatives, which can partially cross the brain-blood barrier, are found in Ginkgo biloba extracts
- P-Tyramine
- N-methylptryramine
- 3-Methoxytyramine
1.2. Noradrenaline-related trace amines¶
1.3. Serotonin-related trace amines¶
- Tryptamine
- N-methylptryptamine
1.4. TAAR agonists¶
- 3-Methoxytyramine (3-MT)
-
Catecholamine neurotransmitter metabolite
- Main metabolite of dopamine in the extracellular space
- Normetanephrine
-
Catecholamine neurotransmitter metabolite
- Dimethylethylamine (DMEA)
- Trimethylamine
-
Catecholamine neurotransmitter metabolite
- Isoamylamine
-
Catecholamine neurotransmitter metabolite
- In particular on mTAAR3 (mouse TAAR-3)
- 3-Iodothyronamine (3IT)
- An endogenous thyroid hormone metabolite
- Putrescine
- Cadaverine
- Possibly agmatine, spermine and spermidine
- N-methylphenylethylamine
- N-methylated metabolites of PEA
- N-methyltyramine
- N-methylated metabolites of TYR
- N,N-Dimethyltryptamine (DMT)
- N-methyl metabolite of TRP
-
Dopamine
- Serotonin
1.5. Sources of trace amines¶
Matured cheeses, fermented meats, red wine, soy products and chocolate have been shown to be enriched with one or more of the aromatic amino acids PEA, TYR and TRP. Seafood showed high levels of gmatine, cadaverine, OCT, PEA, putrescine, spermidine, spermine, TRP and TYR. Tofu also contains high levels of aromatic amino acids. However, aromatic amino acids in food hardly reach the concentration to bind hTAAR, with the exception of hTAAR1 and hTAAR9 in the stomach.
- Decarboxylation of aromatic amino acids occurs in different cell types, so that trace amines can theoretically be formed in these:
- Nerve cells
-
Glial cells
- Blood vessels
- Cells of the gastrointestinal tract
- Kidney
- Liver
- Lung
- Stomach
- Serotonergic AADC, especially in the pylorus
Cells that can produce trace amines by means of aromatic L-amino acid decarboxylase (AADC) were found in the following areas of the brain:
- Raphe nuclei (serotonergic, very strong AADC)
- Pons ventral (serotonergic, very strong AADC)
- Medulla ventral (serotonergic, very strong AADC)
- Mesencephalic reticular formation (dopaminergic, moderate to strong AADC)
-
Substantia nigra (dopaminergic, moderate to strong AADC)
-
VTA (domapinergic, moderate to strong AADC)
-
Locus coeruleus (noradrenergic, moderate to strong AADC)
- Subcoeruleus nuclei (noradrenergic, moderate to strong AADC)
- Median and ventrolateral parts of the intermediate reticular nucleus in the medulla oblongata (noradrenergic / adrenergic, moderate AADC)
- Forebrain: few non-aminergic AADC-positive neurons (D-neurons); these were not detectable in other parts of the human brain.
The enzyme aromatic L-amino acid decarboxylase encoded by the AADC gene occurs in different forms, which cause dopaminergic and serotonergic decarboxylase:
- Dopaminerg: 3,4-dihydroxyphenylalanine decarboxylase
- Serotonerg: 5-hydroxytryptophan decarboxylase
1.6. Regulation of the AADC¶
The aromatic L-amino acid decarboxylase (AADC), by means of which trace amines are produced from precursors, is itself subject to regulatory influences:
- PH value changes
- Denaturation
- Destruction of dopaminergic cells only reduced the dopaminergic 3,4-dihydroxyphenylalanine decarboxylase, not the serotonergic 5-hydroxytryptophan decarboxylase activity, which actually increased. In contrast, another study found that the destruction of both dopaminergic and serotonergic neurons reduced both dopaminergic and serotonergic AADC equally.
- The serotonergic 5-hydroxytryptophan decarboxylase activity and the dopaminergic 3,4-dihydroxyphenylalanine decarboxylase showed divergent activity maxima depending on pH, temperature and substrate concentrations:
- The serotonergic AADC activity:
- In soluble and particulate cell fractions
- Pyridoxal-5-phosphate doubles this
- Pyridoxal-P antagonists hardly affect them
- Serotonergic AADC is potentiated by 3-isobutyl-1-methylxanthine.
- The dopaminergic AADC activity:
- Only in soluble cell fractions
- Distribution corresponded to lactic acid dehydrogenase
- Pyridoxal-5-phosphate increases this by a factor of 20
- Carboxyl scavengers inhibit them completely
1.7. Storage of trace amines¶
Unlike dopamine and noradrenaline, trace amines are not stored in synaptic vesicles. They diffuse from the nerve cell directly and easily through the plasma membranes.
2. Trace amine receptors¶
Trace amine receptors (since 2005: TAAR, Trace amine associated receptor) have been found in the nucleus accumbens and substantia nigra. These are predominantly G-protein-coupled receptors, which therefore increase cAMP when binding to trace amines.
While other animals can have more TAAR (fish have over 100), humans have more than hTAAR:
hTAARs are functional receptors controlled by their own genes, with the exception of hTAAR3, -4 and -7, which are encoded by pseudogenes. In addition, there are said to be hundreds of orphan hTAARs
2.1. HTAAR1 (TA-1, TAR-1, TRAR-1)¶
TAAR1 is most frequently found in the amygdala in the brains of both mice and humans.
TAAR1 are predominantly localized intracellularly, which enables presynaptic and postsynaptic effects
2.1.1. TAAR1 agonists¶
- Attachment affinity:
- Tyramine > β-phenylethylamine > dopamine = octopamine
- Β-PEA > tyramine > tryptamine > synephrine > dopamine > octopamine > serotonin > histamine > noradrenaline.
- S(+)-amphetamine >> P-hydroxymethamphetamine > R(-)-amphetamine > (+)-methamphetamine > R(-)-apomorphine >> 1,2,3,4-tetrahydroisoquinoline > Selegiline > d-LSD > Haloperidol > Chlorpromazine > Phencyclidine > Fluoxetine > Cocaine > Pargyline, Promazine, Clozapine, Risperidone, Clorgyline.
- Tyramine (TYR)
- Β-Phenylethylamine (PEA)
-
Dopamine (DA)
- Octopamine (OCT)
- Amphetamine (AMP)
- Methylphenidate (MPH) is not a TAAR-1 agonist, but could indirectly activate it by blocking the transport of trace amines through monoamine transporters or orphan transporters.
- 3,4-Methylenedioxymethamphetamine (MDMA)
- Lysergic acid diethylamide (LSD)
- Ergoline derivatives
- Ractopamine, an additive in cattle feed for pigs, cattle and turkeys in the USA (not uncritically viewed there and increasingly rarely used); banned in the EU and Russia
- L-isoleucine could be a TAAR1 ligand
- Isoleucine is an amino acid that crosses the blood-brain barrier and is found particularly in poultry, meat, pulses and cheese.
- Phenylethanolamine appears to be a weak TAAR1 ligand
- Mephedrone
- Cathinone derivative
- Weak TAAR1 agonist
- Methylenedioxypyrovalerone
- Cathinone derivative
- Weak TAAR1 agonist
- Some monoamine transporters appear to support the activation of TAAR-1
-
Dopamine transporter (DAT)
- Noradrenaline transporter (NET)
- Serotonin transporter (SERT)
- D2 autoreceptors appear to inhibit TAAR-1
- In the body, TAAR1 regulates nutrient-induced hormone secretion, making TAAR1 a potential therapeutic target in diabetes and obesity
- TAAR1 can regulate immune reactions by controlling the differentiation and activation of leukocytes.
Effect of TAAR agonists.
The selective TAAR1 partial agonist RO5203648 has an antipsychotic, antidepressant and somewhat anxiolytic effect. It increased dopamine in the VTA and serotonin in the raphe nuclei. RO5203648 attenuated addictive behavior and significantly promoted attention, cognitive performance and alertness.
The selective TAAR1 agonist RO5166017 inhibited the firing frequency of dopaminergic neurons in the VTA and serotonergic neurons in the raphe nuclei. The firing frequency of noradrenergic neurons in the locus coeruleus remained unchanged. RO5166017 altered the desensitization rate and agonist potency at 5-HT(1A) receptors in the dorsal raphe nuclei. RO5166017 reduced stress-induced hyperthermia by acting at TAAR1. RO5166017 reduced dopamine-dependent hyperactivity in cocaine-treated, DAT-KO and NMDA antagonist-treated mice.
The partial TAAR1 agonist RO5263397 reduced intravenous self-administration of METH in rodents with chronic exposure to psychostimulants.
2.1.2. TAAR1 antagonists¶
EPPTB (N-(3-ethoxy-phenyl)-4-pyrrolidin-1-yl-3-trifluoromethyl-benzamide)
2.1.3. Occurrence of TAAR1¶
-
Hypothalamus
- Preoptical area
-
VTA
-
Amygdala
-
Dorsal raphe nucleus
- Nucleus tractus solitarii
- Parahippocampal area (rhinal cortices)
- Nucleus tractus solitaire
- Subiculum
- Not in
- Olfactory bulb
- Purkinje cells of the cerebellum
- HTAAR1 (also) occurs in the stomach, particularly in the pylorus
2.1.4. TAAR1 and the dopaminergic system¶
Trace amines and dopamine show complex interactions. TAAR1 regulate dopamine, which is associated with the high expression of TAAR1 in VTA and substantia nigra.
- The trace amines β-PEA and TYR are synthesized in dopaminergic terminals but are not stored in vesicles. β-PEA and TYR diffuse easily through plasma membranes.
- β-PEA and TYR recovery takes place via OCT2
- D2R presynaptic inhibits PEA/TYR synthesis.
- The degradation of dopamine by COMT generates 3-MT (TAAR1 agonist).
- Extracellular 3-M, extracellular PEA and extracellular TYR are agonists of the TAAR1/D2R heteromer complex both presynaptically and postsynaptically.
-
Dopamine appears to induce c-FOS luciferase expression via TAAR1, but only in the presence of DAT
- TAAR1 agonists reduce the firing rate of dopaminergic neurons in the VTA
2.2. HTAAR2 (GPR58)¶
2.3. HTAAR3 (GPR57P)¶
2.4. HTAAR4 (TA2, TA2P, 5-HT4P)¶
2.5. HTAAR5 (PNR)¶
Agonists of hTAAR5:
- Dimethylethylamine (DMEA)
- Trimethylamine (TRA)
- Do not bind to hTAAR5:
- Dimethylamine
- Methylamine
- Trimethylphosphine
- Cyclohexylamine
- N-methylpiperidine
- Pyridine
- Β-Phenylethylamine
- Skatol
- Ethanolamine
- Putrescine
- Isobutylamines
- Dimethylbutylamine
2.6. HTAAR6 (TA4, TRAR4)¶
TAAR-6
* Is associated with schizophrenia and bipolar disorder in humans
* Not G-protein-coupled
* Does not bind to monoamines (unlike TAAR-1)
* Does not occur in monoaminergic brain regions in rhesus monkeys
2.7. HTAAR7 (TA12, TAAR7a and others)¶
Other earlier designations and isoforms are TA15 (TAAR7d), TA14 (TAAR7e), TA13P (TAAR7f), TA9 (TAAR 7g), TA6 (TAAR7h).
2.8. HTAAR8 (TA5, TRAR5, TAR5, GPR102)¶
Other earlier designations and isoforms are TA11 (TAAR8a), TA7 (TAAR8b), TA10 (TAAR8c).
2.9. HTAAR9 (TA3, TRAR3, TAR3)¶
- HTAAR9 is not G-protein-coupled
- HTAAR9 (also) occurs in the stomach
3. Degradation of trace amines¶
3.1. Resumption¶
It is possible that PEA is resumed by the OCT2 transporter.
If this proves to be the case, this would provide an approach for drug interventions.
3.1. Metabolization¶
Trace amines are primarily broken down via MAO-A and MAO-B, and semicarbazide-sensitive amine oxidase (SSAO; also known as vascular adhesion protein-1 (VAP-1) amine oxidase, copper containing 3 (AOC3)). Catabolization via cytochrome P450 seems possible, but is of secondary importance.
4. Interaction dopamine system / trace amine system¶
The dopamine system and the TAAR1 system interact in complex ways:
- TAAR1 is a dopamine and amphetamine receptor that interacts with DAT and D2 receptors. TAAR1 plays an important role in regulating the addiction-related effects of stimulants and the dopaminergic system
- TAAR1 can migrate to the cell surface after heterodimerization with D2R, an effect that promotes D2R signaling, although this occurs through the Gi signal transduction cascade rather than the β-arrestin-2 pathway.
-
Presynaptic D2R activation via the Gi signal transduction cascade leads to inhibition of PEA/TYR synthesis.
-
Dopamine is degraded extracellularly by COMT to 3-MT. 3-MT is a TAAR-1 agonist which, like extracellular PEA and TYR, can activate the TAAR1/D2R heteromer complex at both pre- and postsynaptic membranes.
- There are no interactions between D1R and TAAR1.
The TAAR1 ligands PEA and TYR show indirect sympathomimetic effects similar to those of amphetamine, e.g. reuptake inhibition and displacement of monoamine neurotransmitters from the vesicles. However, this only occurs at very high levels of at least 10 µM (probably 100 times the usual physiological concentrations) or when extremely high doses of more than 25 mg/kg) of PEA/TYR are administered.
Octapamine shows similar indirect sympathomimetic reactions
Various amphetamine derivatives bind to TAAR1, see above.
Unlike amphetamine (and other drugs), PEA does not trigger a conditioned taste aversion reaction.
Graphic from Wikipedia: Dopamine
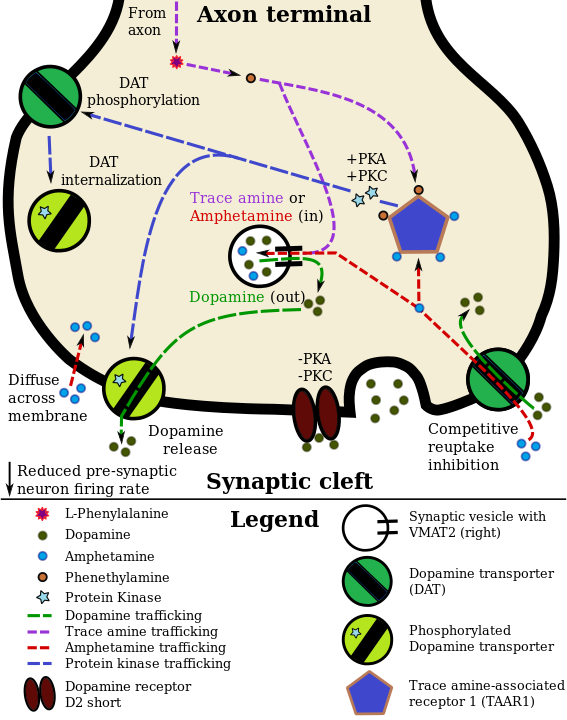
By Seppi333 - Own work, CC BY-SA 3.0, https://commons.wikimedia.org/w/index.php?curid=31186161
Illustration of a dopamine neuron with a colocalized TAAR1 receptor and the effects of a TAAR1 agonist (amphetamine or a trace amine such as phenethylamine) on dopamine reuptake and efflux: Amphetamine enters the presynaptic neuron via membrane or DAT. There it binds to TAAR1 or enters the vesicles via VMAT2. When amphetamine or a trace amine binds to TAAR1, it reduces the firing rate of postsynaptic dopamine neurons (via mechanisms not shown) and triggers signaling by protein kinase A (PKA) and protein kinase C (PKC), leading to DAT phosphorylation. The phosphorylated DAT then either releases dopamine (efflux) or retracts into the presynaptic neuron and ceases transport. When amphetamine or a trace amine enters the synaptic vesicles via VMAT2, dopamine is released into the cytosol (light brown area).
5. Trace amines and ADHD¶
Endogenous trace amines have been associated with ADHD since the mid-1980s.
Some studies found significantly reduced levels of β-phenethylamine (PEA), phenylacetyl acid (PAA), phenylanaline and P-tyrosine in the urine of children with ADHD or ASD, as well as PAA, phenylanaline and tyramine in plasma
MPH and AMP normalize the PEA concentrations in the urine of boys with ADHD.
Another small study found elevated tryptophan blood levels in ADHD-HI correlated with hyperactivity.
A very small study found in ADHD:
- 4-pyridoxic acid (4PA) / tryptophan (TRP) ratio reduced
- Indoxyl sulphate (IND) / tryptophan (TRP) ratio reduced
- Indoxyl sulfate (IND) / kynurenine (KYN) ratio reduced
- TRP (tryptophan) tripled
- KYN (kynurenine) more than doubled
- 3-HOKYN (3-hydroxykynurenine) more than doubled
- KA (kynurenic acid) strongly increased
- IND (indoxyl sulfate) reduced
which indicates a dramatically impaired activity of the pyridoxine-dependent enzymes and a congenital disorder of B6 metabolism (as in epilepsy). The KYN / TRP ratio represents an index of indoleamine 2,3-dioxygenase activity, the enzyme that limits tryptophan degradation. The 3-HOAA / 3-HOKYN ratio is an index of kynureninase activity. MPH appears to increase kynurenic acid and decrease quinolinic acid in plasma in ADHD. MPH did not alter TRP degradation, but created a kind of equilibrium between some of the metabolites detected, but not in the 4PA/TRP, IND/TRP or IND/KYN ratio. Long-term treatment with pyridoxine normalized ADHD symptoms without serious side effects.
Another study found no significant abnormalities in tryptophan, tyrosine or phenylalanine in children with ADHD, although reduced blood levels of these substances tended to correlate with ADHD.
A meta-study found that ADHD:
- higher kynurenine levels (SMD = 0.56), also in drug-naïve children with ADHD (SMD = 0.74)
- reduced kynurenic acid levels (SMD = -0.33), also in drug-naïve children with ADHD (SMD = -0.37)
- increased tryptophan levels in drug-naïve children with ADHD (SMD = 0.31)